Every slope - no matter how gentle - represents a safety risk for men or buildings because under certain circumstances it can give rise to a slide of lesser or greater velocity. Thus one of the duties to be performed by the engineering geologist and geotechnical engineer is to assess the stability of a hillside or slope in order to guard people from injury.
Often it is a very simple matter to determine the stability of a hillside from its appearance and a knowledge of its bed rock; often certain signs will indicate that a slope is moving, albeit slowly; and often enough slides have occurred and caused devastation before the experts have looked into a slope's safety.
Two approaches are usually taken to assess a slope's stability. The first is to conduct a mathematical stability analysis after careful exploration of the subsoil; the findings reflect the momentary degree of stability. The second approach is to install a monitoring instrument that can identify
- the state,
- the mechanism of the movement and
- any time-related change of stability-affecting factors,
providing the basis for effective safeguards.
If a slope’s safety of about 1 or less in smaller sliding masses has been found out mathematically or by control, safety steps will be taken which will increase the safety to a degree as stipulated in our standards.
But often the volume of the moved mass is so big that safeguards are not possible. In such cases the state of the slope will be continuously controlled and in case of imminent danger for men and buildings appropriate precautionary measurements will be taken.
When controlling the state of a slope above all the time-related development of the slide is in the foreground. From the information about the movement’s velocity you can estimate the risk of the slope and if safety measurements will be necessary and which ones.
In 1995 the International Union of Geological Sciences (IUGS) has classified slides according to their velocity (see Tab. 1). These classes enable to reveal about the risk’s degree and to start precautionary measurements.
Tab. 1 Velocity classes of slides (according to IUGS, Working Group on
Landslides, 1995)
Velocity classes |
Description |
Velocity limits |
Value |
VII |
Extremely rapid |
||
----------- |
---------------- |
5 m/s |
5 × 10³ |
VI |
Very rapid |
100 |
|
----------- |
---------------- |
3 m/min |
50 |
V |
Rapid |
100 |
|
----------- |
---------------- |
1.8 m/hour |
0.5 |
IV |
Moderate |
100 |
|
----------- |
---------------- |
13 m/month |
5 × 10‾³ |
III |
Slow |
100 |
|
----------- |
---------------- |
1.6 m/year |
50 × 10‾6 |
II |
Very slow |
100 |
|
----------- |
---------------- |
16 mm/year |
0,5 × 10‾6 |
I |
Extremely |
1) Multiplication factor between lower and higher velocity limit
Often the mechanism of a movement cannot be recognized only by geotechnical exploration, it must be found out by geotechnical measurements. Without knowledge of the movement’s development you cannot make a stability calculation. There are three different mechanisms: (s. Fig. 1):
- Rotational movements,
- translational movements, and
- tilting movements.
Fig. 1 Different movement mechanisms in the development of slope slides
1 Rotation, 2 Translation, 3 Tilting
The use of instruments to monitor a slope can be planned all the better, the more that is known about the stability affecting factors that may be the cause of a slide. The most important of these factors are:
- Changes of slope angle: These can have natural or man-made origins (e. g. cuts in the base caused by water erosion or excavations). An increase of the slope's gradient produces a change of stress in the rock, and the higher shear stresses disturb the conditions of equilibrium. Theoretically the change of stress could be measured, but practically such a measurement is the exception.
- Change of slope height: Vertical erosion or excavation work results in a decrease of horizontal stresses; this leads to a loosening of the rock and the formation of fissures parallel to the slope, making it easy for surface water to penetrate the hillside. The loosening can be very well monitored by displacement measuring instruments.
- Vibrations: Earthquakes, blasting and machine vibrations can disturb a slope's equilibrium as the result of brief changes of rock stresses. Vibrations change the intergranular bond in loess and sand, reducing cohesion. Vibration velocity sensors installed in the slope are suitable for measurement.
- Changes of water content: Precipitation and melt water gets into fissures and generates hydrostatic pressure. The porewater pressure in the soil rises while the shear resistance drops. Precipitation measurements indicate a correlation between increasing slope movements and exceptional precipitation. As the result of electro-osmotic processes, the increased water content results in unequal electric potential on the two sliding face boundaries and hence in a higher risk of sliding. Precipitation water penetrates argillaceous rock particularly easily after long periods of dry weather because the water can easily seep into shrinkage cracks. Groundwater gauges and piezometer are used for measurement.
- Effect of the ground water: Flowing ground water produces seepage pressure, which reduces a slope's stability. Sudden changes of the ground water level result in porewater pressures, which can cause liquefaction of sandy soil. A current of ground water may also wash out soluble grain bonding substances, which results in a reduction of the rock's mechanical properties. In fine sand and silt, flowing ground water washes out the fine grains, which again leads to a decrease of rock strength. Perched ground water exerts considerable pressure on above lying layers and can also lead to destabilisation of the slope.
- Effects of frost: The formation of ice in fissures causes them to open and grow larger, reducing the cohesion of the rock. In clay and clayey sandy soil, the formation of ice lenses results in an increased content of water after melting. Freezing of the surface obstructs the drainage of the slope, causing the ground water level to rise with possible impairment of the slope's stability.
- Weathering: Both mechanical and chemical weathering can reduce the cohesion of the bed rock. The chemical weathering of argillaceous rock through hydration and ion exchange has led to the triggering of many a slide.
- Effect of vegetation: Tree roots may contribute, on the one hand, to the stabilisation of slopes through the absorption of part of the ground water. On the other hand, tree roots have an immense intrinsic effect and result in fissure enlargement.
The geotechnical measurements listed under the particular items are aimed at monitoring and quantifying as many of the above mentioned sources of destabilisation as possible.
The complete description of Geotechnical Measurements on Hillsides and Slopes can also be downloaded here as a pdf.
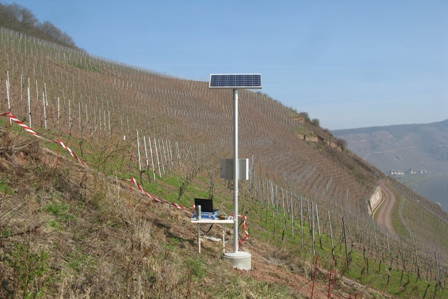